BAERI’s Taylor Bell and a team of researchers have identified the presence of methane in the atmosphere…
Planet Twins and Martian Winds
In this episode we hear from planetary scientist Victoria Hartwick who works with the Mars Climate Modeling Center at NASA Ames. The conversation includes a discussion about Dr. Hartwick’s paper that explores whether wind turbines on Mars could be a viable source of energy for future humans on that planet, then ranges to how clouds form in the highest reaches of the atmosphere, how climate models work, and how planetary climates, including our own here on Earth, are affected by the space environment around them.
Listen here or on Apple Podcasts, Audible, Spotify, or Google Podcasts.
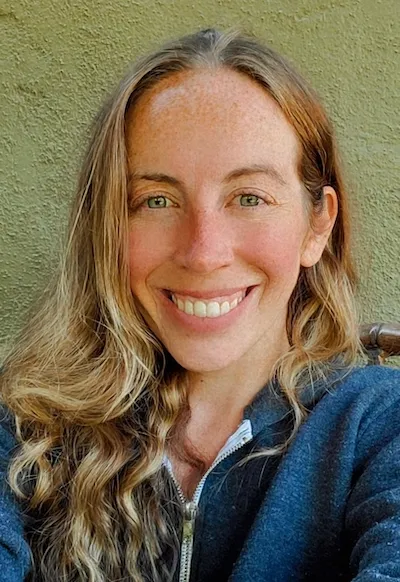
BAERI researcher Dr. Victoria Hartwick. Image: Victoria Hartwick
Quick Glossary
Exoplanet — Any planet outside of our solar system (i.e., a planet revolving around a star that is not our Sun)
Climate model — Computer programs that simulate weather patterns over time
Kelvin — The temperature scale used in exoplanetary science
Ablation — The breaking up of a meteor when it travels through Earth’s atmosphere
Noctilucent clouds — Pearlescent extremely high-altitude clouds visible at dawn and dusk. They are formed on meteoric smoke.
This transcript has been lightly edited for clarity.
Danielle Levin: This is for the Love of Science, a podcast from the Bay Area Environmental Research Institute. I’m Danielle Levin. In this show, we hear directly from the institute’s scientists, engineers and mission specialists about the groundbreaking research they’re doing right now in Earth, environmental, and space sciences, and learn what their work can teach us about Earth and our universe.
In this episode, my colleague and our host, Erin Bregman, speaks with planetary scientist Victoria Hartwick, who works with the Mars Climate Modeling Center at NASA Ames. The conversation begins with a discussion about Dr. Hartwick’s paper that explores whether wind turbines on Mars could be a viable source of energy for future humans on that planet. The interview begins there, but explores topics large and small, from how clouds form in the highest reaches of the atmosphere, to how climate models work and how planetary climates, including our own here on Earth, are affected by the space environment around them.
For listeners less familiar with the scientific terms, we’re starting something new with this episode and including a glossary of terms as part of our show notes on the Bay Area Environmental Research Institute page on Medium.com. On that page, you’ll find our podcast and also articles covering all facets of BAERI: the research, the team, and more. And now on to Erin’s interview with Dr. Victoria Hartwick.
Victoria Hartwick: I’m Dr. Victoria Hartwick. I am an employee at the Bay Area Environmental Research Institute, working with the Mars Climate Modeling Center at NASA Ames Research Center. I guess that the through line of my research has been applying lessons from Earth, or lessons from Mars, to other planetary systems, and using these really advanced tools, global climate models, to ask really fundamental questions about what’s driving climate. And I think there’s a lot of opportunity to explore, to ask fun scientific questions, to just stretch our understanding of how planetary climate works and what it means to be, you know, an evolving climate, or a habitable climate, in the solar system and in our galaxy.
Erin Bregman: I like that that’s kind of how you started, you get to ask interesting science questions. Because one of my questions for you was going to be: how do you get to your science questions? What’s the process for arriving at a question that you want to investigate more?
Victoria: That is a really great question. Science is, I guess I would call it a collaborative process. And the way you get ideas, in some ways it’s really a creative endeavor. You pull in information from all of these researchers, and the state of the art of what they’re working on, and it’s almost an insight moment where you can see how different pieces connect together. And there’s often just that one thing that is missing and you say, “well, what about what about this aspect?” And that’s just the seed of your research project.
This project on wind turbines on Mars came out of that type of process. I did my Ph.D. at University of Colorado, Boulder, in the Atmospheric and Oceanic Sciences Department, so I was the lone planetary scientist in a sea of Earth scientists, some of whom were doing global climate simulations using these global climate models, and others who had more practical applications to very specific questions about the Earth meteorology.
One of my colleagues was an expert on wind turbines for Earth, and her dissertation focused on utilizing global climate model data to predict the available wind power in specific locations across the planet. As she was explaining it, I realized: I have a global climate model of Mars that produces exactly the same data types as she’s discussing. And I wondered, could I just simulate the wind energy and the possible wind power and evaluate it in the same fashion? And so in collaboration with her and a couple other wind energy experts at the University of Colorado, I was able to adapt Earth methodologies for investigating wind power to Mars.
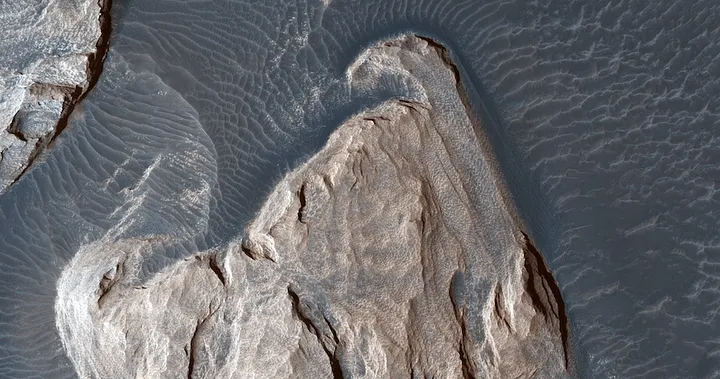
This image was taken by NASA’s Mars Reconnaissance Orbiter in December 2018. The ripples in the Martian sand tell us which way the wind was moving and how it was diverted around these rock formations; Image: NASA/Jet Propulsion Laboratory-Caltech/University of Arizona
Erin: And why hadn’t that really been done before?
Victoria: I think the major reason that not a lot of time had been spent investigating the potential wind power on Mars is because the atmosphere is incredibly thin. The atmosphere on Mars is about 1% the atmospheric density on Earth. So if you had a strong wind on Earth, say, like a 50 mile per hour wind, which would really–you’d be working against it to move, the equivalent force that you’d feel against you on Mars for a 50 mile per hour wind, it’s kind of like a gentle spring breeze. And how wind turbines work is actually the wind moving over the blade starts it spinning. So you need force behind your winds to get your turbines rotating and therefore generate energy. And because Mars’s atmosphere is so thin, even with really strong winds, it was kind of the general consensus that maybe there wasn’t enough power available.
But a lot of those first assumptions were based on wind speed measurements at very particular locations on Mars. We actually have only directly observed the Mars winds at a couple locations across the surface. And many of those locations were actually chosen because we believed that the winds would be low.
Our first landed missions, we don’t really want gusty winds coming through as you try to land your spacecraft. Similarly, we have some measured winds from the Insight Lander, which is a recent mission to Mars, and they needed it to be really quiet so it wouldn’t interrupt the instruments. So they wanted really low winds. So we just assumed that, you know, the winds across the surface, maybe those measurements were representative of the global wind budget.
We found using our global climate model that there are places that are quite windy and can in fact sustain wind energy pretty reliably. In the past, you were relying on these really simple models of Mars or just, like I said, direct observations, and now we have these really sophisticated models that allow you to probe, you know, the entire planet and look at very discrete different properties. And so we had this tool and it allowed us to look at a question that had been put to the side for a bit, I would say.
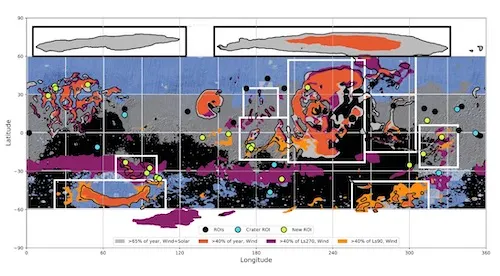
This figure shows proposed regions of interest for wind energy deployment (in boxes) based on the percentage of the year during which wind, or wind in combination with solar, can generate the energy requirements for a human mission on Mars. Gray regions show locations where solar and wind power would produce more than 65% of the energy requirements throughout the entire Mars year; Image: Victoria Hartwick
Erin: Yeah, that’s another great segue, because I’m so interested in these global climate models. Can you talk a little bit about what it is and how it’s made and how you use it?
Victoria: Of course. So global climate models, I like to kind of think of it as like a planet in a box. So it’s a tool, a computer model, or a computer simulation, of the entire planetary system. And these can be of varying complexity. But the most state-of-the-art nowadays can capture really the entire, all the processes that are determining the climate and meteorology on a planet. These often derive from Earth models, and those models are utilized to do things like weather prediction and also to look at, like, long term trends in climate. Starting, I would say, in the maybe ’70s, we started adapting those Earth models to evaluate the climate and weather on different planets.
One of the cool things about planetary science is the physics that drives the climate and weather on earth is the same physics that drives the climate and weather on other planets. So we can utilize what we know about Earth and apply it to other planets.
So we took these really complicated models and started adapting them to other planets. Some things that would be included for Mars would be dust lifted off the surface and moved through the atmosphere, the motion of the winds. And that’s very important for this wind energy paper. But it can get even more complex. You can start looking at cloud formation. You can look at evolution over hundreds of thousands of years. The possibilities are really endless.
Erin: And I imagine there are a whole lot of variables that go into a model like that. Like how many are there?
Victoria: There are so many. Because when you think about anything that you can imagine that would impact the weather in your backyard, there are so many factors that could impact it. And when you’re looking at a planetary scale, you can make this as complicated as you want. Particularly important for Mars and particularly important for the winds on Mars would be things like the local topography. So really accurately matching the topographic maps that we have, looking at if there’s snow anywhere on the surface or changes in the surface thermal properties. So something that would make the surface warmer or cooler. Also, things that are important are how much dust is in the atmosphere because dust is actually absorbing incoming solar radiation and outgoing heat. And that can change how hot the atmosphere is at different levels and that changes the circulation and therefore the wind. So a lot of times what we can do is directly take the topographic map and put it into the model. Or in the case of this study, we used observations of the evolving dust and just, we didn’t simulate it, we just utilized a map and that helps constrain some of the outputs.
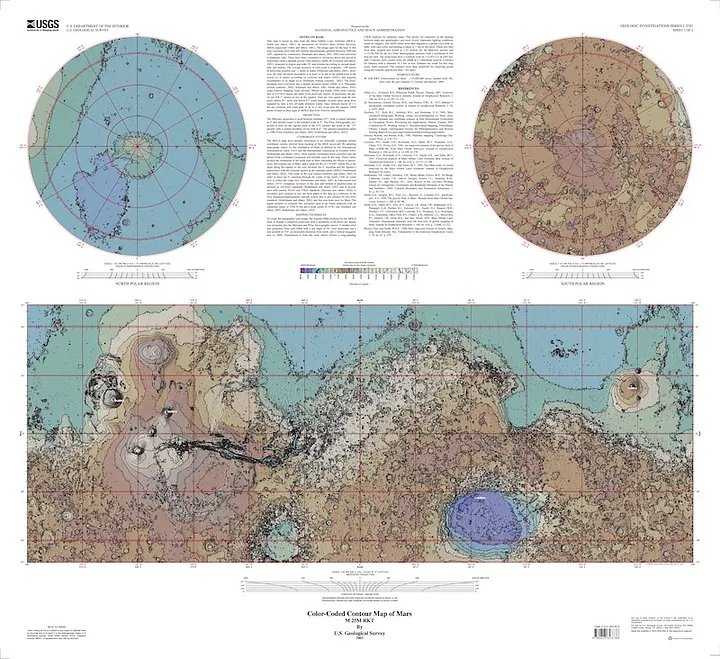
This map of Mars is based on data from the Mars Orbiter Laser Altimeter, an instrument on NASA’s Mars Global Surveyor spacecraft. The image used for the base of this map represents more than 600 million measurements gathered between 1999 and 2001, adjusted for consistency, and converted to planetary radii; Image: U.S. Geological Survey
Erin: And how did you get started down this path?
Victoria: So I started exoplanetary studies…. I saw a hole in some of our current evaluations of exoplanets. We are really concentrated, logically, on trying to find or identify an Earth twin. So something that’s like Earth that could possibly be habitable. That’s very exciting. My work with Mars, present day Mars, made me, I guess I’d say nervous, that it might not be as easy to tell an Earth-like planet from a Mars-like planet that happens to be quite cloudy.
So my work is to take the Mars climate model for present day Mars and move that planet into different orbital configurations and different stellar types and see how the system adapts and changes. So to look at how the dust changes, to look at how the dust and the clouds cooperate together and modify the observed spectrum that we might get.
My first study of this, we just took Mars and shoved it to Earth orbit around the sun and said, “Well, what is this going to look like? What if we had Mars smack in the middle of the habitable zone?” And we were really surprised to see that it got very dusty. But also temperatures raised above 273 kelvin, which is the temperature that we think about when we want liquid water on the surface. So this little rocky planet with a tenuous atmosphere could actually be habitable by that classic constraint. So this was not an expected result. And sort of hints at that there… That we have more to know or more to learn when we think about exoplanet habitability.
Erin: It sounds really fun to be surprised by what a model does.
Victoria: I think that some of my favorite things about being a scientist is, you know, you run your model and you have some idea of what you expect, visualizing the data and pulling through it and you’re like, “What is going on? What does this mean?” It takes a lot to try to parse through it and figure it out and wrap your mind around what this complex planetary system is doing.
Erin: I was looking at another one of your papers, too. I think it was an earlier one about clouds and Mars. Can you talk a little bit about that work too?
Victoria: Yeah, of course. So this was some work I did as a doctoral candidate at the University of Colorado, and I was really interested in these observations we’ve seen of clouds on Mars that are very high up in the atmosphere, above 50 to 70 km. There was a question about how these clouds could form. The basis of that is that clouds don’t like to form just out of nowhere, water likes to condense onto something. And on Mars, that’s mostly dust. But dust is really difficult to mix up to that level in the atmosphere. So we wondered what could be up at that level of the atmosphere, which would act as a site for water to condense on and form clouds.
And this is another example where we look to Earth scientists and some of the work they’ve done to give us insight into what might be happening on Mars. So there’s a type of cloud on Earth called noctilucent clouds, which are these incredibly high altitude clouds. And they actually form on meteoric smoke, which is basically, after tiny, tiny meteors come into the atmosphere, they burn up and that burnt up remnants coagulate and form little particles and clouds form on that material. So I always think that’s just a cool concept that we have clouds forming on meteors, basically, in our atmosphere.
Erin: How often does that happen?
Victoria: They’re really pretty common on Earth. They’re in a very specific area. You’ll see them kind of in the polar regions, very high in the atmosphere where it’s quite cold. And they have this sort of pearlescent color. So sometimes you can see them at dawn and dusk. They’re very pretty, and pretty cool when you know the origin of them.
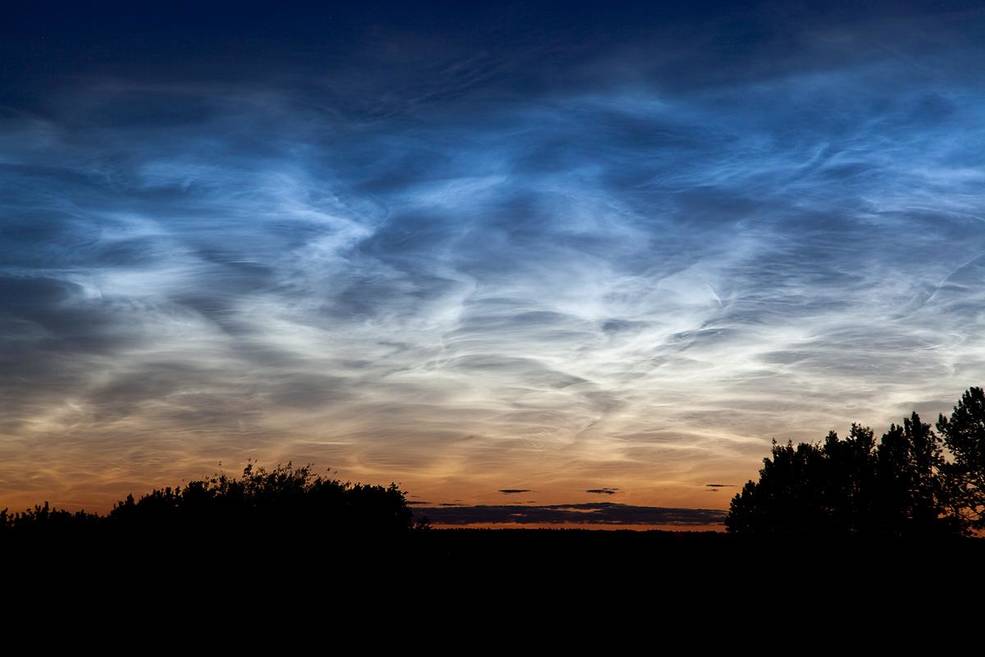
Noctilucent clouds in the sky above Edmonton in Alberta, Canada, on July 2, 2011; Image: NASA/Dave Hughes
Fortuitously, a spacecraft had just arrived at Mars called the MAVEN spacecraft, and it was actually measuring metallic iron layers in the Mars atmosphere, which are the byproducts of meteoric ablation or the burn up of these meteors as they entered the atmosphere. So we had an observational constraint that suggested that this type of process to form clouds could be happening on Mars as well as on Earth. So we utilized the observations from MAVEN and put that into our model and were able to simulate the formation of clouds on this meteoritic smoke material. And we were able to reproduce some of the observations of these really high altitude clouds which were difficult to produce without that source of material at the top of the atmosphere. So that was a cool little thought experiment to see if some of the processes we see on Earth are actually happening on Mars as well.
Erin: Yeah, that’s very cool. And I think it was in a piece about the paper, I’m not sure if it was in the paper itself, you were quoted as saying that we think of these bodies as self-contained planets, but climate isn’t independent of the surrounding solar system. And that, to my mind, a little bit like, Oh, right, of course we think about our planet as contained, but it’s actually part of this bigger thing that’s actually impacting it.
Victoria: Yeah, it’s really easy to think of, you know, our planet as fully impacted by processes that are happening just internal to the planetary system. But really, we’re in an environment, a space environment, so we’re impacted by the Sun, and that one’s a little easier to understand. We’re going through debris fields from the formation of our solar system or from impacts long ago. We’re also being impacted by other planets in our solar system. And for Mars, these meteoric, smoke particles, the formation of clouds can actually have kind of planetary scale climate impacts, which is really interesting in that it’s not just a localized change. It can have a large impact. And this could change earlier on in our solar system history when there’s a lot more just junk hanging out in our solar system still. The impact could have been even bigger.
Erin: I feel like we hear about the Armageddon-style meteor impacts, not the day to day stuff.
Victoria: Yeah, these are much more nice and less threatening, but it’s a lot of material. You know, tons of material per day. My advisor at the time, he told me that you can actually try to collect this. So if you go up to the top of your roof and, you know, get some water down, I think they’re magnetic often, and you can collect some of those like meteoric debris from very, very small… I’ve never tried it, so I don’t know how easy it is, but tons of tons of meteoric material is coming into Earth every day and it just burns up in the atmosphere because it’s very small.
Erin: And you said that planets in the solar system are affecting each other too?
Victoria: Yeah. So one of the most obvious ones is Jupiter. And I like to think of Jupiter as our protective big brother because he sits out in the orbit and his large atmospheric, or gravity reroutes asteroids and material into different directions. And there’s some scientists who believe that Jupiter was really important for the stability of life on Earth because we would have been impacted by a lot more asteroids if Jupiter wasn’t constantly kicking them out of the way. So the whole solar system is in this kind of concert together to change the evolutionary history of our solar system.
Erin: Huh. And where my mind goes is like, okay, if that’s a unit, then like, what’s affecting the solar system and what’s…
Victoria: Yeah, because the solar system is in our galactic neighborhood. It’s moving around through space. It’s amazing to think of. And that’s the rabbit hole as a scientist you start going under, thinking what could impact this? How deep does it go?
Erin: Right. And if you’re looking at something like a climate model that, like at some point you just have to cut off what you’re measuring. How do you decide what you’re going to stop looking at?
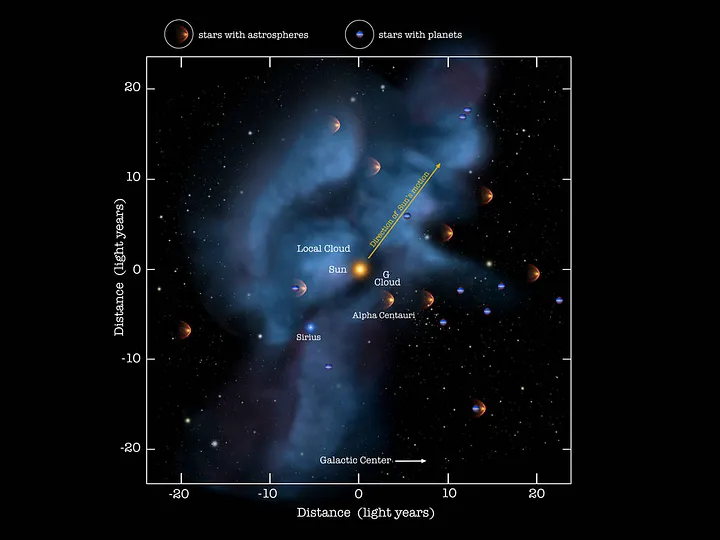
Graphic of the most immediate environment around our Sun, our cosmic neighborhood. Image; NASA/Goddard/Adler/University of Chicago/Wesleyan University
Victoria: I think a lot of times it depends on the question you’re asking. So, for example, if I’m looking at the winds on Mars, the meteoric smoke and the clouds probably won’t have that big of an impact on that system. So maybe that’s not the level of complexity that I add to the model at that point in time. Or, you know, the really long term evolution of the planet. Mars tilts back and forth on its orbit. But when you’re looking at a day to day climate or a day to day wind, we don’t really have to worry about the evolution of the planet on millions of years. So you just make choices based on the specific aspect that you’re looking at. And you try to make informed choices, but we might not always be right. So it’s helpful to keep thinking about it and checking your assumptions.
Erin: Have you encountered instances where you’ve found your assumptions to have been wrong?
Victoria: So often! I think that’s part of the fun of being a scientist. In our most recent paper, for example, on Mars-like exoplanets. We are pretty confident that these systems would get dustier because basically as you move closer in your orbit, the sun is giving more energy just by virtue of you being closer to it. So it’s injecting more energy into your planetary system. So we thought the dynamics would be strengthened and the winds would be stronger and we’d get more dust lifted into the atmosphere.
What we didn’t expect is that dust would accumulate to incredibly high levels and that would have a huge feedback on the dynamics of the system. But also we created a dust greenhouse effect and that was not something I was looking for. It was not something I expected. It wasn’t something I thought to investigate. Because when we think of greenhouse gases or the greenhouse effect on Earth, we’re really conditioned to think about gases, CO2, methane, maybe like sulfurs from volcanoes. But you don’t really think of like a mineral dust lifted from a desert as forming a greenhouse. But on this exo-Mars, there was enough dust in the atmosphere that was actually raising the surface temperatures by tens of kelvins, and in this case, raising it above the freezing point of water. So that was really the determining point of making this potentially habitable is having a dust greenhouse effect.
The fundamental question, I guess, in terms of exoplanets and in terms of what we call arid or Mars like exoplanets, which are just planets that have a limited amount of water, so we’re not talking about oceans, is at the top levels: could these planets be habitable, and what do they look like when we stare at them with a telescope? Can we identify them in the first place? Can we pinpoint signatures of habitability, and can we discriminate them from other types of planets? But at the basis it’s, you know, when we think of planets, what are the constraints of habitability and how far out of our understanding of Earth can we get and still be habitable.
One of the things I think is most interesting, and I mentioned this earlier and I think people don’t always understand, is that planetary science, the processes that happen are driven by the same physics everywhere. So the reason why winds blow on Earth are the same reasons that winds blow on Mars. So just because you’re thinking about a different planet doesn’t mean, you know, you’re in a completely different environment or something completely foreign. We can apply lessons that we’ve learned from different planets sort of back and forth. I also think one of the things that is really exciting about planetary science, and in particular exoplanetary science, is the opportunity to think out of the box. In some ways, these fields are really creative fields that you think about fundamental science questions in creative ways.
Erin: What does science mean to you?
Victoria: Science to me is about attempting to understand the world around us to the best of our meager capabilities as humans, and to sort of remove the curtain from the wonder of the universe. You’ve got me waxing poetic right now, but you can just look outside. And there’s so many questions about: Why is this happening? What makes things work the way they do? And science is our attempt to start answering some of those questions and use all the tools that we’ve developed as human beings over time to try to answer some of these really fundamental questions about the universe.
I don’t know that I can answer that. I can probe, you know, answer the tiny question, and each scientist we just add, we can contribute that little bit. And as a group, we can maybe start figuring things out.
Danielle: Thank you to Victoria Hartwick. Our music is by Danny Clay. You can learn more about Victoria and her work at victoriahartwick.wixsite.com/research. To find that link, this episode’s glossary, and more about the Bay Area Environmental Research Institute, visit our page on medium.com. From all of us at BAERI, thank you for listening and see you next time.