BAERI’s Taylor Bell and a team of researchers have identified the presence of methane in the atmosphere…
The researchers ready for the James Webb Space Telescope
Part II: An interview with Jesse Bregman
In this two-part series we’ll meet two researchers who will soon be working with data from the recently launched James Webb Space Telescope. In this episode, Part II, I speak with Jesse Bregman, an astrophysicist who has spent his 50 year career at NASA Ames, and has been part of some pretty amazing leaps in infrared astronomy during that time. The conversation ranges from the backyard telescope he and his brother built, to how astrochemistry research could lead us to an understanding of the origins of life on Earth.
Listen here or on Apple Podcasts, Audible, Spotify, or Google Podcasts.
This transcript has been lightly edited for clarity.
BAERI: This is for the Love of Science, a podcast from the Bay Area Environmental Research Institute. I’m Erin Bregman. In this show, we hear directly from the institute’s scientists, engineers, and mission specialists about the groundbreaking research they’re doing right now in Earth, environmental, and space sciences, and learn about what their work can teach us about our Earth and our universe.
Welcome to our second of two interviews with researchers who will soon be using data from the recently launched James Webb Space Telescope. If you missed part one, you can still go back and listen to my conversation with early career astronomer Taylor Bell about his work categorizing exoplanets.
I am very excited to share this interview with you as part two, with Jesse Bregman, an astrophysicist who spent his 50 year career at NASA’s Ames, been part of some pretty amazing leaps in infrared astronomy during that time, and who also happens to be my dad. Our conversation ranges from the backyard telescope he and his brother built, to how astrochemistry research could lead us to an understanding of the origins of life on earth.
JESSE BREGMAN: Well, I did write myself a bunch of notes.
BAERI: Okay, well, how would you like to introduce yourself?
JESSE: All right. So, actually we need to talk about that. What do you mean by that?
BAERI: You know how when somebody starts on the podcast, they’re like, ‘What’s your name? Where do you work? What are you doing?’
JESSE: Oh. So I am Jesse Bregman, I am an astronomer slash astrophysicist working on astrochemistry. I study large molecules in space.
BAERI: And are those the polycyclic aromatic hydrocarbons?
JESSE: Those are the polycyclic aromatic hydrocarbons.
BAERI: So what are they and why do you study them?
JESSE: Ah, let me look at my notes. They are large carbon based molecules made up of hexagonal arrangements of carbon, kind of like looking at a chicken wire, and hydrogen atoms on the periphery of those carbon structures. So we study them because they can tell us interesting things about the environments they inhabit, because they are quite abundant, they’re bright, so you can see them, and they’re easily identified from their infrared spectrum. Spectrum changed a little depending on the environment they’re in, which is how you can determine what that environment actually is. They are the most abundant multi-atom molecules in the universe, and they must have been very common on the surface of the early Earth. And they form the central structure of chlorophyll.
BAERI: When you say they’re the most abundant, what does that mean? Like, how abundant is a most abundant molecule?
JESSE: The most abundant atom in the universe is hydrogen. And then helium is the next one. And then you get into carbon, oxygen, and nitrogen, which are about a ten to the minus four, so, one 10,000th as abundant as hydrogen. The abundance of carbon in PAHs is somewhere around 15 to 20% of all the carbon available in the universe. So that’s what I mean, they’re very abundant.
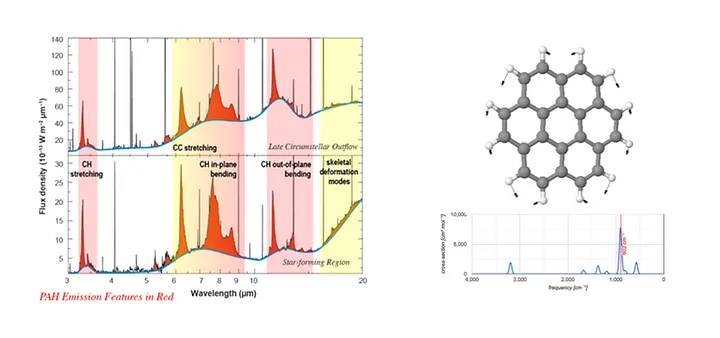
Left: The prominent emission features due to Polycyclic Aromatic Hydrocarbon (PAH) molecules in the infrared spectra of two astronomical objects. Adapted from E. Peeters. Right: Visualization of the vibrations of the active fundamental modes in coronene; Image: NASA
BAERI: How did you get started studying them?
JESSE: So when I was a graduate student, there were these new strange emission bands that were discovered in the infrared. They were discovered in planetary nebulae, which are very old, kind of dying stars. No one knew what they were, and so we started to look at them in detail to try to figure that out. And we didn’t identify them as PAHs, since up to that time, only very simple molecules had been discovered in space. And so when I was looking to try to figure this out, I only looked at molecules that had maybe three or four or five atoms in them. PAHs, the smallest one, has about ten carbon atoms. So it actually took an organic chemist, a physical chemist, to figure out that these emission bands were from these polycyclic aromatics.
BAERI: So these are super abundant, but it was unknown that they existed?
JESSE: That’s right. This was 1972, it was unknown that they existed at that time.
BAERI: Why was that still unknown?
JESSE: This has to do with the advance of technology. You find these things through infrared spectroscopy and the early infrared spectrometers used in astronomy came about in the 1960s. People were looking at stars primarily because they were bright things, and then started looking at nebulae. You had to find one that was bright enough that you could actually get a good signal off of it. And one day, some people down at Caltech found these very strange emission bands in a couple of planetary nebulae. Up until that time, they had never been seen. This was probably around 1970.
BAERI: What makes them strange?
JESSE: They’re quite broad, and they’re very bright. And there was nothing that you could identify that caused them.
BAERI: So people were looking at this and confused?
JESSE: People were looking at this as, ‘Oh, great, we’ve got another puzzle here! This should be really interesting to try to figure out! I wonder what they are?’ And like I said, you know, it took until the mid to late seventies until Lou Allamandola actually came up with this idea of these aromatic molecules. And the first response to that was, ‘You’ve got to be kidding. Those are huge. How can they possibly be there?’ But as it turned out, that’s what they were.
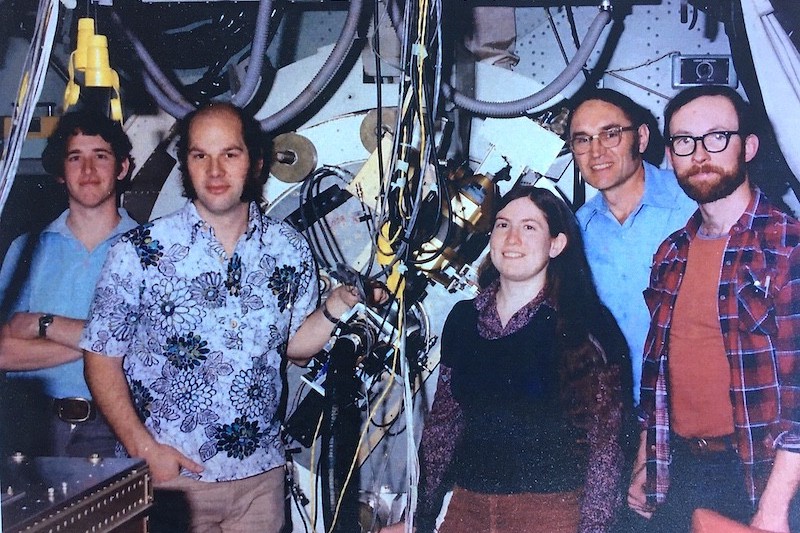
Bregman and colleagues with their infrared spectrometer (gold, at center) installed on the Kuiper Airborne Observatory telescope, circa 1980. From left to right: Dan Lester (University of Texas), Jesse Bregman (NASA Ames), Harriet Dinerstein (University of Texas), Fred Witteborn (NASA Ames), and Harold Crean (technician); Image: Jesse Bregman
BAERI: Why did it seem impossible that something that huge would be there?
JESSE: Well, so to form a molecule, you have to put atoms together. And the density in space is so low that two carbon atoms getting together happens quite rarely. You actually have to form these around stars. And there’s a phase in stars where they’re losing their envelopes and the density is still low, but it’s millions of times higher than it is in interstellar space. And so that’s where these things were forming. And just that whole concept, that whole idea of that’s what was going on was really foreign at that point. This was a new idea. So you know, it was that leap into a new frontier, essentially, like, ‘Oh my goodness, there’s things going on here we never even conceived of.’
BAERI: And that’s when you started grad school?
JESSE: That was my early years in grad school. Yeah, I started grad school in 1971. This was part way through, and the identification of PAHs didn’t happen until after I was out of grad school.
So this is an interesting thing, because this tells you how science works. So this one band at 11.2 microns fit calcium oxide very well, or magnesium oxide, one of the oxides. Which sort of made sense. I mean, there’s, you know, magnesium oxide has two atoms to it. But then you look at the rest of the spectrum and say, ‘Okay, well, if that’s magnesium oxide at 11 microns, then there should be a very strong band there over at seven microns.’ And the researchers who went and looked for that did not find it. And so you could then rule out magnesium oxide. They found an incredibly strong band at 7.7 microns. And another one at 3.3 microns. And those fit the aromatic spectrum very, very well. So that’s how you have to investigate whether your ideas are correct or not by checking against other things that you should see.
BAERI: Where did that early information come from? And that was a new technology? What was the new technology?
JESSE: So the infrared spectrometers were the new technology, and the first data came from people at Caltech, so they were probably using the 200 inch, on Palomar. We had in those days a spectrometer that Dave Rank had built, and I had done the computer programs that were digitizing the data so we could actually see what the instrument was producing and plotting it as spectra.
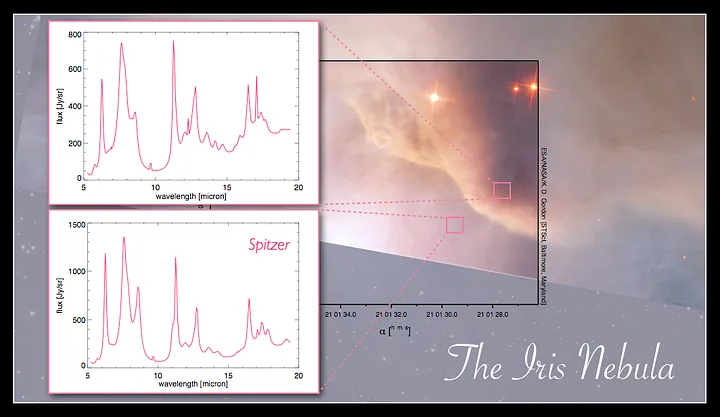
Two examples of the PAH infrared signature from two positions in the Iris nebula; Image: astrochem.org/pahdb
BAERI: And when you say computer programming, what did that mean at that point in computers?
JESSE: Well, what I did was known as machine language programming. And so you’re working down at the fairly low level of the microprocessors to turn on analog to digital converters that are attached to the, actually attached to the spectrometers and controlling switches, essentially, to select which detector you wanted, and then reading them through the A to D converters and saving that into some memory in a computer that had a total of 8,000 bytes of memory. 8,000. Not eight gigabytes, eight kilobytes of memory. Then using a teletype, that was the interface to the… You know, there were no keyboards, essentially, it was a keyboard with a printer. It looked like a modification of a typewriter, essentially, with motors in it. Made a horrendous noise all night long. And then you would just sit there and command with the teletype, or with switches on a panel, you could tell the computer to start taking data. And you would do that for hours on end.
BAERI: Well, I guess that connects directly to my next question, which is how much does a telescope impact what you can then understand?
JESSE: Enormously. The infrared is an interesting part of the spectrum because everything around you is glowing in the infrared. So observing from a telescope on the ground in the infrared is like working in the visible with the lights on all the time. And the sun up. So there’s not a lot of contrast there. And so that’s the background you’re trying to fight. So if you could put that telescope in space, which has been done numerous times, it is really cold and it’s really dark up there, even in the infrared. And so the sensitivity is enormously better. I mean, I don’t know what the number is, but it’s got to be tens of thousands of times more sensitive up there. And so something like the Spitzer Space Telescope was just amazing at what it could do. And that’s still the best infrared data ever taken, and will be until James Webb starts taking its data as well.
BAERI: Do you expect James Webb data to be so much better again that it’s just a giant leap, or is it more incremental than ground based to Spitzer?
JESSE: It will be different. The advantage to the Webb telescope is that it has much higher spatial resolution, so you can see variations in much smaller dimensions. And also it has higher spectral resolution. So you’ll be able to see more spectral details than was possible with Spitzer. And it has a wider wavelength range. So you’ll be able to operate in wavelengths from 1 to 5 microns where there was no data at all from Spitzer. So any time you increase the capabilities like that, you will find things that you did not imagine existed.
Which is funny because when you write a proposal to get time, you have to tell everybody what you’re going to find. You can’t say, ‘Well, I don’t know what I’m going to find, but let’s go look!’ That doesn’t work. So you make up a story and you have a plan. And so we’re going to look at a particular wavelength range, 1 to 5 microns, where we expect to see the spectral signatures from particular molecules of interest.
So to me, the most interesting thing we’re going to do has nothing… Well, it does have a little bit to do with PAHs. So, deuterium is an atom that is also known as heavy hydrogen. So it has a proton and a neutron in the nucleus. All the deuterium in the universe was made in the Big Bang, and has been slowly being destroyed ever since. Because when you put deuterium into a star, the temperature is hot enough, it reacts with other atoms and basically goes away. So if you look around the universe, an important thing to do then is to measure the amount of deuterium, and that tells you the conditions in the Big Bang. All the way back to the beginning.
If you look around in the galaxy, you find that there is not a single number for the amount of deuterium. In some places, it looks like there’s not enough deuterium. And one of the thoughts is that the deuterium has been captured into PAHs, because deuterium is a little less reactive chemically than hydrogen, and so once it gets into a PAH, it sticks better. So what we’re trying to do then is try to measure the deuterium to hydrogen ratio in PAHs and see in fact, whether there is a lot of deuterium hidden in the PAHs. And ideally, how much is hidden in the PAHs. Now that can be added back to what you see in the gas phase in the same direction and say, ‘Aha, here is what the total amount of deuterium was when it was formed in the early seconds of the universe.’
BAERI: And is that connected to star formation and life cycle in general, or is that more just straight backwards looking?
JESSE: Well, it’s sort of connected. One of the questions you could ask is how many times the material in our galaxy has gone through a star? So in a star’s life cycle… How are we going to put this? Do you want me to go through the life cycle of a star?
BAERI: Yeah! I mean, if there’s like a five minute version of it or less, you don’t need to get into the weeds weeds.
JESSE: Oh yeah, it’s easy.
BAERI: Yeah, I think that would be really useful.
JESSE: Okay. A star is born, it lives, it dies.
BAERI: That’s it, huh?
JESSE: That’s it.
BAERI: Nothing else to it?
JESSE: So a star starts to form when a region of space consisting of gas and dust collapses under its own gravitational force. And then it gets hot in the center because the gravity is converted to heat, and it can get hot enough to then convert hydrogen to helium in a fusion reaction. And we define an average star as, strangely enough, the mass of our Sun. Which turns out it’s not a bad average star. And then the star has enough hydrogen in the center that it lasts about 10 billion years. After 10 billion years, all the hydrogen is converted to helium, and the star core starts to collapse under its own gravitational weight. And the temperature goes up, and it gets to the point where helium starts to fuse into carbon, and that lasts another billion years or so. Well, when this core collapses, the atmosphere of the star also expands. It becomes a red giant, because there’s more energy being generated faster at that point. Then for a star like our Sun, the process then goes to where the atmosphere leaves, it’s blown away as what’s called a planetary nebula, which has nothing to do with planets, and then what’s left behind is a white dwarf. A white dwarf has the mass about the mass of the Sun, but about the size of the Earth. So it’s really dense.
If you have a more massive star, something that’s like, say, ten solar masses, ten times the mass of the Sun, then more collapses happen in the core. And until you convert whatever it is there at the time to iron, iron cannot be fused, and so the star core completely collapses, releases an enormous amount of energy, and you end up with a supernova. And in that supernova explosion, all the elements heavier than iron are formed. Which is really a pretty amazing thing. And that gets blown into space, and then the cycle starts again.
The second time this happens, of course, you have now other heavier elements. Because originally you started out only with essentially hydrogen, helium, and lithium, and the lithium is destroyed in the star fairly early on. So one of the questions is: How many times has this happened in the 13 and a half billion years of our universe? Well, you know that some fraction of the material is deuterium, and the deuterium is destroyed when it goes into a star. So by measuring the current amount of deuterium and calculating the originally formed amount of deuterium and looking at the difference, you can actually tell how many times the interstellar medium has been cycled through a star. How many cycles have actually happened.
BAERI: And do you know that yet for the things you’re looking at, or is that one of the things that you’ll learn?
JESSE: That’s what we hope to learn.
BAERI: What generation is our Sun?
JESSE: I think it’s third generation, mostly. Is that right? Yeah. The sun is about 5 billion years old. More or less. So there was kind of 10 billion ish years… well, 8 billion years before that. It depends on what star you’re talking about, right? The heavier stars that go supernova only live for like 20 million years. They are 10,000 times as bright as our Sun, and so they burn out faster. It’s an interesting question. I don’t know the answer. I’m sure you could look that up.
BAERI: It’s just something that had never occurred to me before. Like, how many life cycles old is our star?
JESSE: How many cycles do you have to go through to get to the material balance that we have now? That’s a very interesting question.
BAERI: And does that then… I imagine that affects the material that’s in the planets around the star?
JESSE: Oh yeah. Right.
BAERI: We haven’t really talked much about how you sidled into astrophysics or astronomy in the first place. Can you talk a little bit about that?
JESSE: So when I was probably seven years old, my brother and I talked my dad into buying us, with all the money we had in the world, we donated so he would buy us a little telescope. I think it cost $10 at the time in the mid fifties. And we would take it out and look at things. You know, we looked at the moon and we looked at the planets. We looked at airplanes flying by. As time went on, when we were teenagers, we built a bigger telescope. My brother ground a mirror and we would take that out and look at the planets once again. With that, we could see the polar caps on Mars. We thought it was pretty spectacular to be able to see that.
When I was an undergraduate, I was a physics major. For work one summer, I worked in a Van de Graaff accelerator lab in the physics department, and I found this to be incredibly boring. So I started taking astronomy classes. And the astronomers I worked with there were really interesting. I should say they made the research really interesting. I thought, ‘Well, this is what I want to do. This is way better than physics.’ So I started doing astronomy.
BAERI: And so did your brother.
JESSE: And so did my brother. Right.
BAERI: I didn’t know that you guys built your own telescope. Joel ground a mirror?
JESSE: He did.
BAERI: How do you even learn how to do that?
JESSE: Oh, you order a kit from Edmond Scientific and they have instructions. Then you have to figure out if you have a good surface or not. And we could never quite do that. But we saw an ad in Sky Telescope one day for a company that would figure the mirror. Which means, you know, doing the final polishing, and getting the right curvature, and coat it for $25. And we’d already put like 120 hours into this work. And we looked at each other and we go, ‘Let’s send it off.’ So we spent our money and they made a really nice mirror, which I still have. A little six inch telescope mirror. I don’t use it, but I still have it. And that was a really fine telescope, I must say. It took us probably a year. To do that.
BAERI: And the sky was dark enough in your backyard that you could actually see things?
JESSE: Well, you can see the plants from the middle of the city. We didn’t really have a good understanding in those days of what to look at. I have a much better understanding now about what’s interesting.
BAERI: I would hope so. Is there anything else that we haven’t talked about that you want to mention?
JESSE: I have my definition here of: What does science mean to you?
BAERI: Yeah, what does science mean to you?
JESSE: Well, to me, it’s the pursuit of knowledge and understanding and truth.
BAERI: The pursuit of knowledge and understanding of truth, not the arrival at?
JESSE: Well, arriving is nice. But I know that it will be temporary. I mean, there’s some foundational work that doesn’t change much. But for the most part, if you’re at the cutting edge of research, what you’re doing is adding on to the knowledge that has already existed, and the understanding that already exists, and then somebody else, maybe you, maybe somebody else, will take that to another level. Hopefully.
So if you want the ultimate ‘Why do you want to do this,’ it comes back to life on the Earth. For me, anyhow. We were talking about how a star forms out of this cloud of dust and gas. Well in a similar fashion, that’s how the planets formed as well. We’re the leftover garbage that was circling the Sun, and accumulated into these planets. And so what was that made out of? Well, obviously, there’s rocks and a lot of water out there. But the other things that fell onto the surface of the Earth, among the things that fell on the surface of the Earth, were these complex PAHs, these complex molecules.
You’re evolving on the surface of the Earth, or life is evolving, or something’s evolving that eventually turns into life. And what do you make that up from if you’re the master assembler? Well, you look around and you just grab the things that exist around you. And one of the things that exists around you are these polycyclic aromatics. Hmm. I wonder what I can use that for? Well, they are used in all kinds of ways in life. And so knowing what your chemicals are that you’re starting with to form life, might inform you in some way about the way life originally formed. And so ideally, in the long term, it would be great if we could take an inventory of the abundances of molecules in space in regions that are similar to what it looked like when our solar system formed, and say, ‘Ah ha, there we are. There’s our starting mixture of materials and we’ve gotten to where we are today.’
BAERI: So, origin of life story too.
JESSE: It’s an origin of life story as well. That’s right.
BAERI: I didn’t realize that.
JESSE: Well, that’s a long ways away with a lot of connecting the dots and a lot of research and-
BAERI: A few careers down the road.
JESSE: Yeah, I mean, that brings up… But there are people who work on early origins and how you form really protocells. One of the interesting things that came up with some of the research that was done in the laboratory at Ames, these were people who are looking at mixtures of molecules, including PAHs, and they would irradiate these mixtures with ultraviolet light, or with electrons, and then they would go through a series of stages — these were at very cold temperatures, like ten degrees Kelvin. And then they would warm them up, and they’d watch what the spectrum did as they warmed up, because the materials become more reactive with each other as they get warmer, and you have real chemistry going on.
They noticed that when they were at room temperature and they opened up their system to clean it off, there was always this brown stuff, or yellow stuff, on their surfaces that they had been using to take spectra of the condensates. One of the molecules that was left over, they just did a little experiment one day and dropped some water on it. And under the microscope, they could see that little spheres formed, these little vesicles. So the molecules that were in this residual were such that they were hydrophobic, I guess. They would go away from water. And the way that they went as far away from water as they could was to form little closed cells. Little closed shells. So you go, ‘Wow, is that a protocell?’ Well, maybe. Maybe that is. So it’s kind of neat that you could get there from simple chemistry that probably went on on the Earth every day.
BAERI: Thank you to Jesse Bregman. Our music is by Danny Clay. If you’d like to learn more about Jesse’s work, you can go to researchgate.net/profile/bregman-jesse. You can also find that link in our show notes. That’s it for this episode. See you next time.
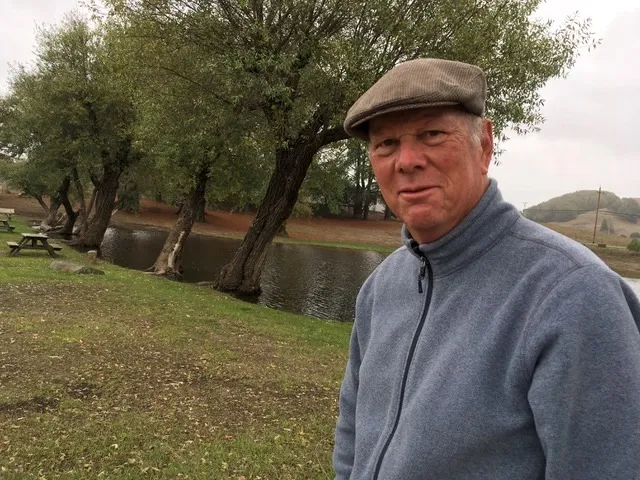
Jesse Bregman